Seismic Monitoring and Protection for Critical Infrastructure
TECHNICAL ARTICLE
26th February 2019
For Immediate Release
SEISMIC MONITORING AND PROTECTION FOR CRITICAL INFRASTRUCTURE
Following the Fukushima incident in 2011 industry has placed a renewed focus on risk mitigation from extraordinary events caused by nature. The various regulatory bodies around the world have gone back to basics to consider not only the design basis for operational sites but also revisit the risk analysis, the accident management strategy and the periodic safety review policy. Considering that many nuclear power stations, oil and gas terminals and critical facilities are located on the coast, particularly true for the UK, the risk of flooding from both seismic events and severe weather has been put in to particular focus. Of significant importance has been the review of methodology used to derive the seismic hazard and how that hazard has been mitigated through the design process and present day operational systems. The operational monitoring of seismic vibration for both structures and equipment plays an important role in providing automatic shutdown protection and the recording of seismic events for post analysis. This article discusses the latest trends in seismic monitoring and protection systems, technologies adopted and current best practice driven by these enhanced risk management demands.
Condition Monitoring Specialists SENSONICS experience suggests no two facilities are the same in terms of their approach to seismic monitoring and protection. Some sites utilise data from the national network of geophysical instruments, whilst others implement independent monitoring and shutdown on each critical plant item. In each case, the derivation of the required seismic monitoring and protection strategy must meet with the safety case and provide appropriate risk mitigation.
The structural effects to be expected at a site from an earthquake result from the vibration induced by the event, classified in terms of seismic response spectra. This defines the ground acceleration magnitude versus frequency, typically over a range of 0.1Hz to 100Hz.
Two such types of spectra are specified, the Operational Basis Earthquake (OBE) and the Design Basis Earthquake (DBE), based on a predicted worst case seismic event within a specified period of time (for example OBE may be specified within 100 years). Secondary response spectra are derived from the ground accelerations through modelling to predict the response of each structure and each level within that structure. Typically a plant will allocate several seismic categories for specifying the design requirements and assess according to the safety class. For example the highest or most stringent category will demand the equipment or process be tested to the DBE level plus a margin (for example +40% is recommended in IEEE- 344, Standard for Seismic Qualification of Equipment for Nuclear power generating Stations), since the process must still remain operable to the design basis even if other less critical plant processes may have failed above the OBE level. Any earthquake above the OBE level may result in the plant being shutdown and to remain shutdown until post analysis / inspection has determined the plant is safe to continue operations.
The challenge is to design and construct in a cost effective manner to meet with the seismic categorisation and to provide sufficient design margin. It may not be possible for all equipment or processes through either analysis or testing to meet with its categorisation fully and this is where independent seismic monitoring systems can be utilised to provide detection of the OBE event and to bring the process to a safe state. Not only must these monitoring systems be robust to seismic events but they also need to exhibit high levels of availability beyond the DBE magnitude event to maintain a valid alarm function. In combination with the seismic requirements various safety standards are applied to obtain a stated availability, with EN IEC 61508 being the most common approach. Adherence to such a standard provides a stated system reliability and availability whilst at the same time providing an understanding of the systematic failures and ensuring compliance with the 61508 life cycle model.
The starting point with any seismic monitoring design is the sensor. And it is important to recognise there is a clear technical difference between the types of sensors that are used for seismic protection and those used for geophysical earthquake monitoring.
Geophysical seismic monitoring utilise broadband magnet & moving coil (electrodynamic) sensor arrangements capable of measuring micro g acceleration events with sinusoidal periods of over 100 seconds. Strong motion sensors on the other hand for seismic protection applications only need to provide a resolution down to 1mg and a response to 10 seconds; whilst historically electrodynamic sensors have been used, nowadays for these applications piezoelectric based accelerometers are preferred as they match the technical requirement closely and provide higher reliability as they have no moving parts.
A trend in vibration monitoring is the adoption of MEMS (Micro Electro-Mechanical Systems) devices in a wide range of sensing applications. These devices offer an excellent low frequency response and exhibit the required dynamic range for strong motion seismic monitoring. MEMS devices have been widely used in civil engineering applications since the 1990’s, their relative low cost and small size suite applications where many measurement points are required on structures for a limited period of time. However, adoption of this technology has been slow for the seismic protection market, where stated reliability and maintainability are the key requirements. MEMS are a fast moving technology and with limited application experience, selection of a specific sensor may prove difficult to maintain in future years as a result of obsolescence.
Significant earthquake events are few and far between, so how do we verify an installed strong motion sensor is working correctly, when for most of the time there is nothing to measure? This situation is exacerbated by the sensor installation which is normally difficult to access. With broadband seismometers it is common to have a secondary coil arrangement which can be excited and therefore stimulate movement of the mass to verify calibration without physical shaking. Sensonics have incorporated a similar mechanism in to its piezo electric based seismic sensors to ensure the measuring element is operating to the correct sensitivity. This self-test feature is a critical requirement which will become apparent when we look at the overall system design.
It is common to utilise redundant sensor configurations in the overall monitoring system concept; refer to figure 1 for a detailed functional block diagram of a modern day seismic monitoring and protection system.
Three separate physical locations are monitored with triaxial sensors capable of measuring acceleration in the three orthogonal axes. The acceleration of each sensor is processed by a trip amplifier with the overall triaxial unit performing a one out of three (1oo3) logic operation to derive the location OBE alarm. The trip alarms from each location are fed back to the central control panel which performs a subsequent two out of three logic operation to determine the final trip result. In this example the voting logic is also redundant to enhance reliability and maintainability. The final element of the system is connected to the specific plant circuit breakers or to the emergency shutdown system to complete the safety loop.
For redundancy, a simple one out of two (1oo2) system will usually meet with the reliability requirements, in fact demonstrating a higher reliability than the 2oo3 system. However, this system configuration offers no protection against spurious trips which can result from mechanical interference or sensor failure. Two out of two (2oo2) is an alternative option that can be considered, however on failure of a channel the system defaults to a 1oo1 system, whilst the 2oo3 option on channel failure can revert to either 2oo2 or 1oo2, both of which are preferred over 1oo1, making the 2oo3 system the norm for critical protection applications.
Combine these channels with dual voting arrangements and the sensor inbuilt test function results in a system design that can be fully proof tested whilst on line, maximizing the availability of the system. Each voting circuit can be isolated and tested in turn through signal injection of each sensor, a critical aspect of the system performance being the sensor will still respond to an real seismic event even whilst under test.
The avoidance of SMART devices within the protection loop also eases the analysis burden to meet with the safety requirements and is the preferred solution for most clients. Separating the protection and event recording functions is a logical step which enables the latest technologies and features to be utilised for the seismic waveform recording without impacting on the protection safety case.
Use of proven technologies in combination with measurement redundancy tends to be the industrial norm for modern day applications; with self-testing features and spurious trip performance being of particular importance in relation to the automatic shutdown systems.
Adopting this best practice has become standard for new installations and should also be considered for obsolete seismic monitoring equipment on existing sites. A stated and demonstrated reliability, minimal spurious trip occurrence, full measurement loop proof testing, maximum design life and maintainability combined with a low demand and high integrity shutdown system is now the expected norm. Typical nuclear industry applications include, fuel handling, reactor structural monitoring, waste processing and the monitoring of crane equipment, while for the oil and gas industry applications include LNG processing, extraction platforms and gas shut-off valves. ENDS.
Further details at:
https://www.sensonics.co.uk/products/seismic_monitoring/structural_monitoring.html
Contact: Russell King, Sensonics Ltd, Berkhamsted, Hertfordshire, UK.
Tel: +44 (0) 1442 876833. Email: sales@sensonics.co.uk www.sensonics.co.uk
Technical Article issued for Sensonics Ltd by:
Andrew Harvey – Harvey Communications
Tel: +44 (0) 1342 714447 Email: admin@harveycomms.co.uk www.harveycomms.co.uk
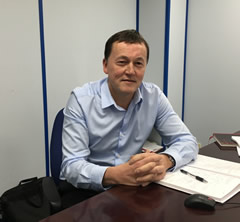